ESG Economist - Direct Air versus Direct Ocean CO2 Capture
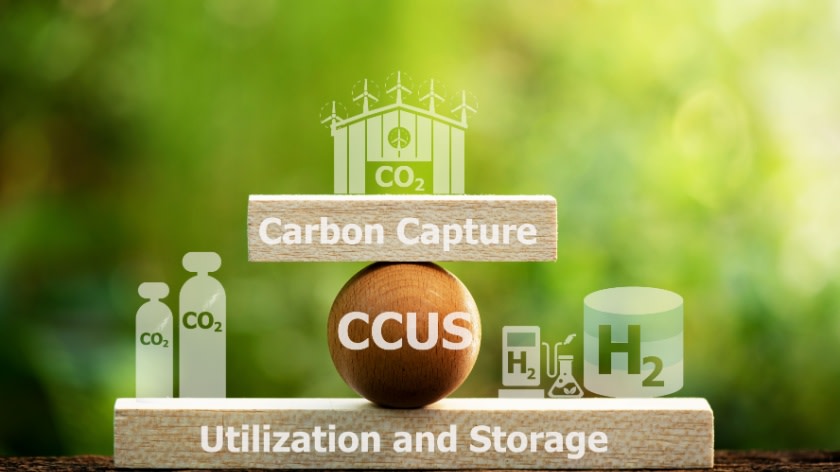
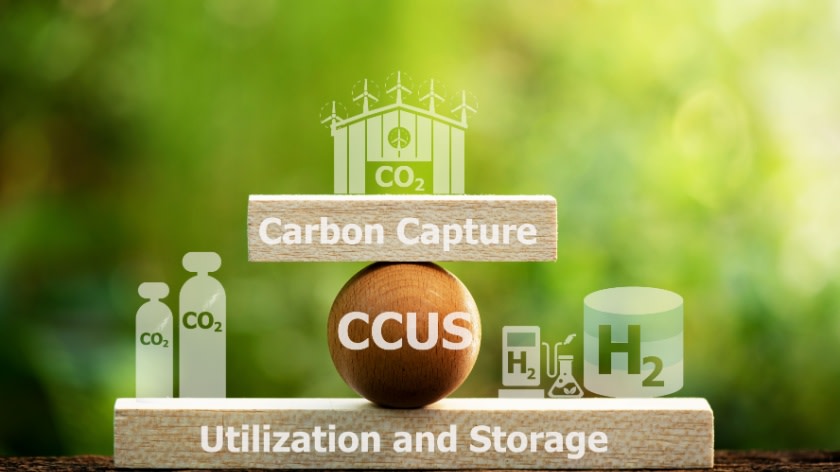
Recently we published an ESG economist about the carbon cycle. There are different places to store carbon such as in the lithosphere, which is the crust and uppermost solid mantle (including fossil fuel reserves), in the soil, in plants, in the ocean and in the atmosphere. But to limit climate change we would need to limit the carbon stored in the atmosphere. According to the Global Carbon Budget there are only 65 Gt, 160 Gt and 305 Gt of carbon left for the 1.5°C, 1.7°C and 2.0°C pathways. This translates into a CO2 budget of 238 Gt CO2, 586 Gt CO2 and 1,117 Gt CO2 for the 1.5°C, 1.7°C and 2.0°C pathways, respectively. As there is only limited availability especially for the 1.5°C scenario, there is a need for technologies that remove carbon from the atmosphere and store it. In recent years we have published several notes on Carbon Capture Storage Utilisation and carbon sequestration geoengineering. In this publication we focus on two technologies, namely, direct air capture and the relatively novel technology direct ocean capture. Direct air capture removes CO2 from the atmosphere and direct ocean capture removes CO2 from the ocean. In the next sections, we compare these technologies and look at their pros and cons. We end with a conclusion.
Various technologies are designed to capture carbon dioxide (CO2) as part of efforts to achieve net zero emissions by 2050
Two methods of CO2 capture are Direct Air Capture (DAC) and Direct Ocean Capture (DOC)
Direct Air Capture (DAC) is expensive because the concentration of CO2 in the atmosphere is relatively low, making the process less efficient
Additionally, DAC is limited in scale, capturing less CO2 compared to the volume of annual emissions.
Electrochemical Direct Ocean Capture (eDOC) is an innovative yet costly technology
Despite its high costs, eDOC has a greater potential to capture more CO2 than Direct Air Capture (DAC)
Direct Air Capture
Direct air capture is a technology that removes CO2 from the atmosphere for storage (see more ) or utilization (see more ). The graphs above show the International Energy Agency (IEA) CO2 emissions and direct air capture under three different scenarios.
The green line represents the Net Zero scenario by 2050, the yellow line represents the Announced Pledges Scenario (APS) and the dark line regards the Stated Policies Scenario (STEPS). In all these scenarios until 2030 the Direct Air Capture capacity is still insignificant compared to the total CO2 emissions globally. After 2030 the role of direct air capture is increasing in absolute and relative terms because global emissions are declining in the Net Zero Scenario. In the other two scenarios, the role of direct air capture is limited as the captured CO2 from the atmosphere remains less than 1% of the total annual CO2 emissions. According to IPCC between 100-1,000 GtCO2 will need to be removed from the atmosphere by the end of the century to limit warming below 1.5°C by direct air capture and other removal technologies.
Besides the fact that there is limited capacity, there is another disadvantage from relying on direct air capture. It is more expensive compared to Carbon Capture and Storage (CCS) as it is very energy intensive. This is mainly because of the low concentration of CO2 in the atmosphere compared to the concentration of CO2 in a flue gas for CCS. However, one major advantage is that direct air capture installations can be placed anywhere, for example, close to renewable energy installations. According to Bloomberg NEF the costs of this technology will decline over time from more 664 USD per tonne in 2024 to 113 USD per tonne in 2050. This is considerably above the current EU ETS prices of 65 EUR per tonne (71 USD per tonne). So, this technology will only become affordable when carbon prices rise above the costs.
Direct Ocean Capture
Next to direct air capture there is a novel technology called direct ocean capture. Before we dive deeper into this technology, it is worth explaining better the behaviour of CO2 in the ocean. That will help explain how direct ocean capture works. In our “ESG Economist: Important dynamics in the carbon cycle” (see more ) we explained how carbon is stored in the ocean. This is a complex dynamic. Oceans store 40-50 times more carbon than the atmosphere. This is because CO2 is dissolved in water and becomes part of dissolved inorganic carbon. This process continues until there is an equilibrium. Dissolved inorganic carbon consists of CO2 in aqueous form, bicarbonate and carbonate with an equilibrium ratio is 1:88:11. This coincides with a pH level of 8. So, a large majority of CO2 taken up by the ocean turns into bicarbonate and there is only a relatively small part of CO2 in aqueous form. Due to this, oceans absorb substantially more CO2 than the atmosphere. The pH of the water determines this ratio. A low pH (more acid) results in more CO2 in aqueous form while with a higher pH there are more carbonate ions. The other equilibrium is the concentration of CO2 in the ocean compared to CO2 in the atmosphere. If the concentration of CO2 in seawater becomes lower than the concentration of the atmosphere, then oceans take CO2 from the atmosphere.
There are several technologies that aim to have oceans taking up more CO2 from the atmosphere. The technology we focus on in this publication is the electrochemical direct ocean capture or eDOC. eDOC is using and changing the abovementioned equilibriums. There are different ways to do this but the methods that avoid pollution to the marine system are preferred (see more ). This technology takes the surface seawater, runs it through an installation and alters its pH. It can substantially lower the pH to 4 (acid) or increase the pH to 10. What happens when the pH of the seawater is altered? At a pH of 4 the equilibrium between CO2 aqueous, bicarbonate and carbonate changes in favour or CO2 aqueous. Then a large concentration of CO2 is filtered out of the water resulting in lower CO2 concentration in the ocean compared to the atmosphere. As a result, the ocean is taking CO2 from the atmosphere again. The captured CO2 can be stored.
At a pH of 10 the equilibrium between CO2 aqueous, bicarbonate and carbonate changes in favour of carbonate. At the presence of calcium and other minerals, bicarbonate and carbonate are mineralized into calcium carbonate. Shells and coral reefs are made of calcium carbonate. So carbon is stored in these shells and reefs but to produce these shells and reefs there is CO2 respiration. When the species die, shells sink to the bottom of the ocean and are dissolved. In this process CO2 is taken from the water.
This technology looks promising but there are downsides to this technology. It is a relative novel technology, and it is not yet scaled. The technical readiness (TRL) of direct ocean capture is now still a pilot to demonstration or TRL 4-6. In addition (renewable) electricity is needed for this technology to work. Lastly this technology also needs iridium and platinum depending on the prototype. The costs are close to that of direct air capture in the case of a co-location with a desalination plant, however, the costs are substantially higher for a stand-alone plant.
Conclusion
There are several technologies that capture CO2 in order to help our quest to reach net zero by 2050. In this note, we focussed on two technologies that capture CO2 namely Direct Air Capture and Direct Ocean Capture. Direct Air Capture is already well known but the costs are still high due to low concentration of CO2 in the atmosphere and its scale is limited compared to the annual CO2 emissions. Then there is electrochemical direct ocean capture technology, which is still a novel technology, whose costs are currently very high. Both technologies need a substantially higher CO2 price to make them attractive. Direct Ocean Capture could be more successful because it takes advantage of the large carbon storage capacity of the ocean (40-50 more than the atmosphere). In comparison, the CO2 captured via the latter technology is much higher than the CO2 captured under the former one.