How to store CO2?
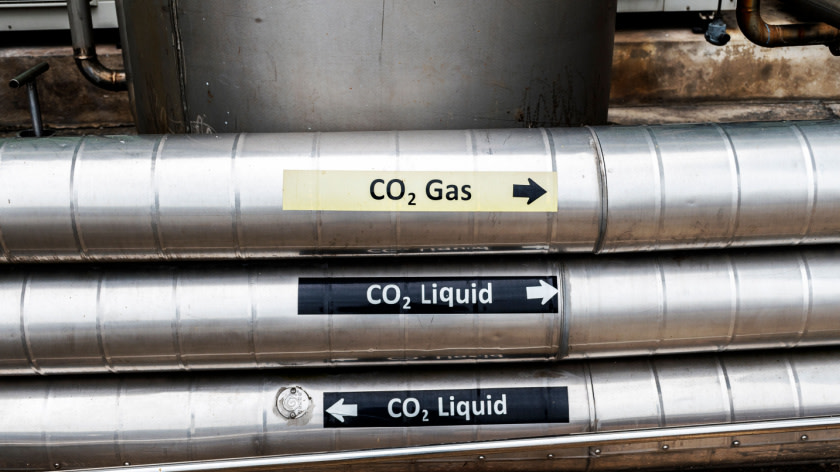
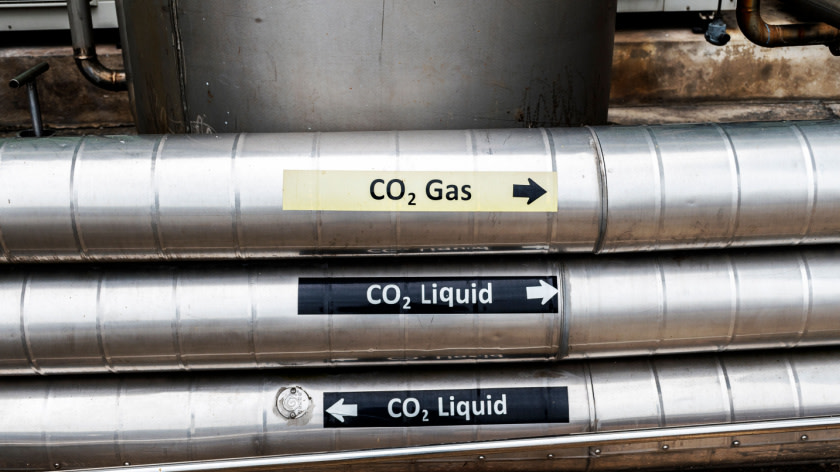
We have done a trilogy on CCS. Our first publication was on the technologies and techniques to capture carbon. Then we focussed on how to transport CO2. In this SustainaWeekly the central question is how to store CO2? We answer what kind of state CO2 needs to be in order to store it. But also in what geological sinks CO2 can be stored and what trapping mechanisms are used. In the future we will also focus on CO2 utilization, direct carbon capture and mobile carbon capture.
Geologic or a biologic deposits can store CO2
Possible geologic deposits are oil and gas reservoirs, saline formations, unmineable coal seams, organic-rich shales and basalt formations
But the CO2 needs to be trapped in the deposit. There are four mechanisms to do that
Costs to store CO2 are dependent on geologic characteristics; scale; and monitoring, financial, and other modelling assumptions
Introduction
Carbon storage means to store the captured carbon in a deposit. Carbon storage is also called sequestration. There are two forms of sequestration namely biologic carbon sequestration and geologic carbon sequestration. Biologic sequestration refers to storage of atmospheric carbon in vegetation, soils, woody products and aquatic environments. Geologic carbon sequestration is the process of storing CO2 in underground geologic formations. In this note we focus on geologic carbon sequestration. CO2 can be stored underground as a supercritical fluid (see our note on ). The main advantage of storing CO2 in the supercritical condition is that the required storage volume is substantially less than if the CO2 were at “standard” (room)-pressure conditions. Temperature naturally increases with depth in the Earth’s crust, as does the pressure of the fluids (brine, oil, or gas) in the formations. At depths below about 800 meters (about 2,600 feet), the natural temperature and fluid pressures are in excess of the critical point of CO2 for most places on Earth. This means that CO2 injected at this depth or deeper will remain in the supercritical condition given the temperatures and pressures present (see ).
Geological sinks
Sequestration technologies deposit captured CO2 in geological formations or through mineralization in reactive rocks. Geologic sequestration is a proven method of underground carbon dioxide (CO2) storage whereby CO2 is injected deep underground into deep rock formations for long-term storage. The major benefit of geological storage of CO2 is that it offers long-term isolation from the atmosphere via permanent trapping of CO2 in the reservoir’s porous medium. The cap rocks are often tight shale rocks with low permeability. One important property of a rock in the presence of two non-miscible fluids (here CO2 and formation brine or salty water) is the preference of one fluid over another to be in contact with the rock's surface, a property called wettability. Wettability directly impacts the flow of injected CO2 in the subsurface and will stay trapped in the rock. The wettability of a system controls the flow and trapping efficiency during the storage of CO2 in geological formations There are five potential geological sinks for permanent CO2 storage: active or depleted oil and gas formations (sandstones/carbonates), deep saline aquifer formations, unmineable coal seams, organic-rich shales and basalt formations.
Oil and gas reservoirs
Oil and natural gas reservoirs are ideal geologic storage sites because they have held hydrocarbons for thousands to millions of years and have conditions suitable for CO2 storage. Injecting CO2 can also enhance oil production by pushing fluids towards producing wells through a process called enhanced oil recovery (EOR). Hydrocarbon reservoirs have limited storage capacity but there is a natural trap by caprock sealing.
Saline formations
Saline formations are porous formations filled with brine, or salty water, and span large volumes deep underground. Carbon capture and storage (CCS) focuses on formations that contain brine with total dissolved solids (TDS) levels greater than 10,000 parts per million total dissolved solids. Studies show that saline formations have the largest potential volume for storing CO2 around the world.
Coal seams
Coal that is considered unmineable because of geologic, technological, and economic factors (typically too deep, too thin, or lacking the internal continuity to be economically mined) may still serve as locations to store CO2. To be considered for CO2 storage, the ideal coal seam must have sufficient permeability and be considered unmineable. Coal seams may also contain methane (CH4), which can be produced in conjunction with CO2 injection in a process called enhanced coal bed methane (ECBM) recovery. In coal seams, the injected CO2 can be chemically trapped by being adsorbed (or adhered) to the surface of the coal while CH4 is released and produced. Adsorption is the adhesion of atoms, ions or molecules from a gas, liquid or dissolved solid to a surface. This trapping mechanism allows for permanent storage of CO2. Coal seams are often closer to emission sites but injection is extremely difficult.
Shales
Some shales have similar properties to coal, having the ability to trap CO2 through adsorption (adherence to the surface), subsequently releasing methane and making them potentially attractive for storage. Despite their low fluid conductivity, CO2 can diffuse into and react with shale minerals and potentially migrate to fill shale . Organic-rich shales trap a significant amount of CO2 permanently.
Basalt
Basalt is a type of formation that was deposited when large flows of lava spread from volcanoes cooled and then solidified. Over time, thick layers of basalt were built up (with other formation types often layered in between). The chemical and physical properties of these basalts, as well as the other formation types in between basalt layers, make them good candidates for CO2 storage systems. Basalt allows injected CO2 to react with magnesium and calcium in the basalt to form the stable carbonate mineral forms of calcite and dolomite. This mineralization process is promising to be a valuable tool for CCS because the mineralization process permanently locks carbon in the solid mineral structure, thereby permanently trapping the CO2 (see ). Injecting CO2 into porous basalt rocks has been identified as one of the most promising techniques for CO2 storage. There are three factors to take into account. The first factor is the porosity of the rock, which affects the surface area available for the CO2 to interact and be stored. Another vital factor for efficient geological storage of CO2 is the permeability of the formation, which greatly affects the injection rates. Higher permeability allows for high injection rates. The third factor is the reactivity of the rock. These rocks comprise minerals that include divalent metal cations. If the cations are unstable, the reaction will occur faster because the cations are far from the equilibrium constant. This results in faster dissolution rates in the more reactive rock and rapid mineralization (see ). However, there are some challenges. For a start large water consumption is required for the mineralization process. In addition basalt rock formations are heterogeneous because of their original depositional environment. Moreover, it can take thousands of years from start to finish for all the carbon to mineralize and at any point a shift in the rocks can cause some carbon to escape.
Trapping mechanism
The CO2 is securely sequestered in the deposits through four trapping mechanism (see ). These mechanism prevent upward migration and leakage of CO2.
Structural trapping
Residual trapping
Solubility trapping
Mineral trapping
Structural trapping
Structural trapping is the physical trapping of CO2 in the rock and is the mechanism that traps the greatest amount of CO2. Structural trapping means the sealing of CO2 by a caprock. Once injected, the supercritical CO2 can be more buoyant than other liquids present in the surrounding pore space. Therefore, the CO2 will migrate upwards through the porous rocks until it reaches (and is trapped by) an impermeable layer of seal rock. Caprock is an extremely low permeable rock which prevents the flow of buoyant CO2 plume. This rock layer can be hundreds of feet thick and keeps CO₂ trapped securely. The rock layers and faults within and above the storage formation where the CO2 is injected act as seals, preventing CO2 from moving out of the storage formation.
Residual trapping
Residual trapping refers to the CO2 that remains trapped in the pore space between the rock grains as the CO2 plume migrates through the rock. The existing porous rock acts like a rigid sponge. When supercritical CO2 is injected into the formation, it displaces the existing fluid as it moves through the porous rock. As the CO2 continues to move, small portions of the CO2 can be left behind as disconnected, or residual, droplets in the pore spaces which are essentially immobile, just like water in a sponge. These reservoirs are not large. When residual trapping occurs, the CO2 is contained.
Solubility trapping
In solubility trapping a portion of the injected CO2 will dissolve into the brine water that is present in the pore spaces within the rock. The CO2 interacts with the brine water, leading to solubility trapping. At the CO2/brine water interface, some of the CO2 molecules dissolve into the brine water within the rock’s pore space. Some of that dissolved CO2 then combines with available hydrogen atoms to form HCO3-. This dissolution increases the density of brines, causing them to sink even lower in the formation—reducing upward migration of CO2 (see ).
Mineral trapping
With mineral trapping the CO₂ interacts with minerals present in the rock formation at the molecular level via a series of geochemical reactions. These reactions result in the formation of new, solid and stable carbonate minerals. The CO₂ actually becomes part of the rock.
Storage costs
The vast majority of CO2 storage potential worldwide is in onshore and offshore saline aquifers (USGS, 2013). The cost of CO2 storage is very site dependent because the geologic characteristics vary from site to site and injection, labor, drilling, capital, and other costs vary regionally. Similar to offshore pipelines, offshore CO2 storage is generally more expensive than onshore storage. For CO2 storage in saline aquifers, various types of wells must be drilled (exploration, injection, and monitoring) which comprise a large share of the overall storage cost.
Previous studies have suggested the cost of CO2 storage in depleted oil and gas fields is lower than in saline aquifers because the oil and gas fields have already been surveyed and offer the potential to reuse existing infrastructure. However, the infrastructure need to be able to transport CO2 which has other characteristics than other gasses or liquids. So the integrity of the infrastructure needs to be verified and monitored. There are costs involved in checking the infrastructure and there are also costs involved in adjusting the infrastructure for CO2 transport. These costs may negate any cost savings, . CO2 storage costs hinge on three major sources of variability: geologic characteristics; scale (i.e., amount of CO2 stored); and monitoring, financial, and other modelling assumptions. A handful of geologic parameters are primary determinants of whether a reservoir is favourable for CO2 storage: permeability, thickness, depth, porosity, and lateral continuity (see ).