The GHG reduction challenge for commercial vehicles
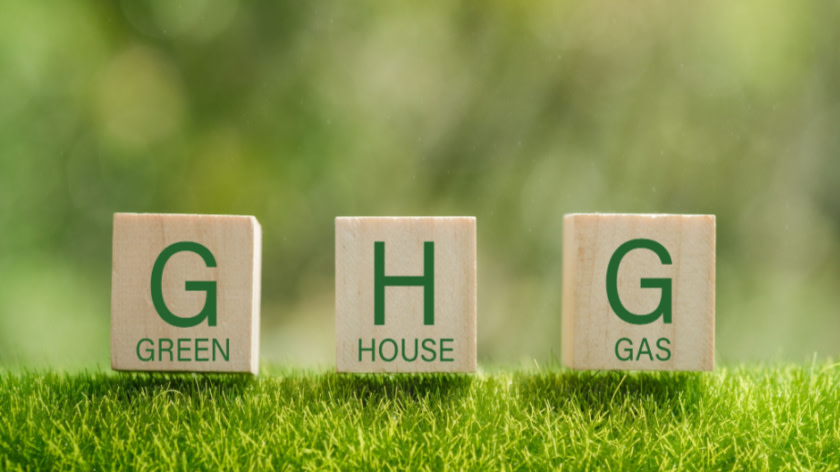
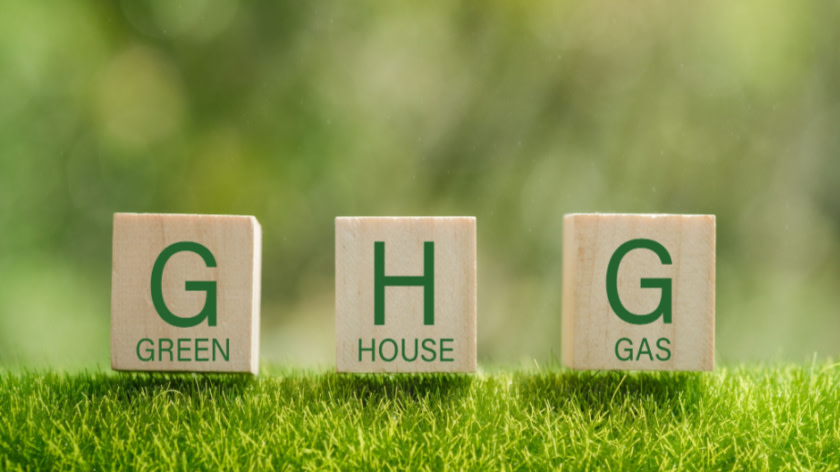
Commercial vehicles account for almost half of the emissions of the mobility sector so will be a key part of the transition. For commercial vehicles to meet emission reduction targets there are three main challenges: the range and freight challenge, refuelling infrastructure challenge and the charging infrastructure challenge.
Commercial vehicles account for 46% of the GHG emissions of the mobility sector so they need to contribute their share to reduce emissions
The targets are very clear for buses, vans and state-owned vehicles. The new proposed targets for heavy duty vehicles are ambitious if approved.
For commercial vehicles to meet emission reduction targets there are three main challenges: the range and freight challenge, refuelling infrastructure challenge and the charging infrastructure challenge
Introduction
The Netherlands has set the goal to reduce emissions of greenhouse gasses (GHG) by at least 55% (with policy aimed at 60%) by 2030 and to be net zero in 2050. The mobility sector accounted for 18% of the total greenhouse gas emissions in 2021. To reduce GHG emissions by the mobility sector the government has set ambitious targets for this sector. The sector specific target for mobility is to reduce GHG emissions from 31 Megaton in 2021 to 21 Megaton in 2030 or a reduction of 10 Megaton. Road transport is the biggest emitter in the mobility sector. It is responsible for around 85% of the total emissions of the mobility sector. Passenger cars account for 50% of emissions of the mobility sector. Commercial vehicles account for 46% of the GHG emissions. The contribution of passenger cars was already discussed in our Sustainaweekly of 6 February. Commercial vehicles also need to contribute their share to reduce emissions. In this report we focus on commercial vehicles.
We start with providing a brief overview of the different types of commercial vehicle and how many there are on the road. We then go on to provide more detail about the Dutch government policy and the EU policy to reduce emissions in the sector. Finally, we assess the numerous challenges commercial vehicles face to reduce emissions.
Commercial vehicles
Commercial vehicles consist of light-duty vehicles (LDV), medium-duty vehicles (MDV), heavy-duty vehicles (HDV), buses and construction traffic.
At the end of 2019, the global fleet of cars stood at 1.083 billion, while the fleet of commercial vehicles stood at 406 million (source ). These commercial vehicles mainly have internal combustion engines that use fossil fuels. At the end of 2021 the total stock of electric trucks stood at 66,000, representing of just 0.1% of the fleet. According to the IEA, the fleet of electric buses was 670,000 at the end of 2021 or 4% of the global bus fleet.
In the EU, in 2021 there were 29.5 million light-commercial vehicles (up to 3.5 tonnes) on the road and 6.4 million medium and heavy commercial vehicles and 714.000 busses. In the Netherlands there were around 158,000 trucks, 1.06 million vans and 9,316 busses on the road in 2021 (). Heavy duty vehicles are responsible for 28% of the CO2 emissions from road transport in the EU, but they are only 2% of the vehicles (FT).
Policy to reduce emissions from mobility
In this section we discuss the emission targets and policy announced by the Dutch government and the European Commission (EC) to reduce emissions for the mobility sector. We start with the Netherlands followed by that of the EC.
The Netherlands
The government target for the mobility sector is to reduce greenhouse gas emissions to 21 Megaton in 2030, this means a reduction of 10 Megaton. Every sub-category needs to contribute its share. The largest share is for personal cars. We focussed on this in the Sustainaweekly of 6 February. In this report we focus on commercial vehicles. Heavy duty trucks emit around 6.2 Megaton and need to reduce 2 Megaton by 2030. Light duty vehicles/vans emit around 3.7 Megaton and need to reduce 1.2 Megaton by 2030. Buses account for 0.9 Megaton emissions and need to reduce at least 0.3 Megaton (see tables above). The government has set clear policy targets for personal cars, busses and vehicles used by the government. Moreover there are also rules concerning city logistics. Below we set out an overview of the targets in place.
From 2025, all new buses used in public transportations should be zero-emission buses and they must use regional produced renewable energy. From 2030 all buses used in public transportation should be zero-emission buses. In addition, all vehicles used by the state should be zero emission as well in 2030. In 2019, the European Clean vehicles directive (CVD) was adopted and in 2021 this directive was implemented in the Netherlands as the ‘Regeling bevordering schone wegvoertuigen’ (Pianoo, 2021b). The regulation obliges government agencies to tender a minimum percentage of clean vehicles in European tenders for vehicles and transport services. All modes of road transport are covered by the regulation. This regulation is forecast to reduce emissions by 0.4 Mton in 2025 (source CE Delft). Finally, there are also new developments on city logistics. In 2025, in the Netherlands, 30 to 40 municipalities have zero emissions zones for trucks and vans. The expected CO2 reduction of this measure is 1 Megaton (Klimaatnota 2022). If we take the announced policy into account the Netherlands could be able to reduce 9 Megaton GHG in 2030 (see table above).
The European Commission
The European Commission has also targets and policy in place to reduce emissions from road mobility (see table below).
On 22 December 2022, the European Council and Parliament agreed to create a new, separate emissions trading system for the buildings and road transport sector and fuels for additional sectors, in order to ensure cost-efficient emissions reductions in these sectors that have been difficult to decarbonise so far. The new system will apply to distributors that supply fuels to the buildings, road transport and certain other sectors. The co-legislators agreed that the system will start in 2027. The linear reduction factor is 5.43% from 2028. So from 2028 the number of allowances will decrease by 5.43%. On top of that they will auction upfront in 2027 30% of the total volume of the number of allowances to secure a smooth transition path. In case the energy prices will be exceptionally high, the start of the new ETS will be delayed until 2028. Once the system has started if the price of allowances exceeds € 45 over a certain period of time, additional allowances will be released increasing the supply on the market.
On 14 February 2023, the European Commission proposed ambitious new CO2 emissions targets for new heavy-duty vehicles (HDV) from 2030 onwards compared to 2019 levels. The proposed targets for new HDV are as follow: 45% emissions reductions from 2030, 65% emissions reductions from 2035 and 90% emissions reductions from 2040. So HDV should from 2030 on emit 45% less CO2 emissions compared to 2019 levels. Emissions in the HDV sector have been increasing year-on-year since 2014 (except 2020). Especially in the freight sector emissions are increasing rapidly. These vehicles run for 99% on ICE largely fuelled by diesel. City busses will have to be zero emissions by 2030 according to the plans (source ).
For commercial vehicles to meet emission reduction targets there are three main challenges: the range and freight challenge, refuelling infrastructure challenge and the charging infrastructure challenge. Below we discuss these issues in more detail.
Range and freight challenge
There are several ways to reduce emissions from vehicles. First, a larger share of the vehicle fleet being made up of zero-emission vehicles. Second, using fossil free biofuel and renewables instead of fossil fuels in internal combustion engines. Third, a combination of both. In this section we focus on viable options for zero-emission vehicles. This can be either a battery electric vehicle or a fuel cell battery electric vehicle. For shorter ranges battery-electric vehicles are a good solution. However for longer ranges and/or transporting heavy cargo, battery electric vehicles may not be up to the challenge. This is because the longer the range, the larger and the heavier the battery would need to be, given the current state of technology. This will increase load for the commercial vehicle.
Currently battery electric trucks on the market have a range up to 300 km with an average weight of 30 tonnes. According to Eurostat statistics, around . 33% of trucks cover daily distances of 500 km or less. In Europe, drivers are legally required to stop for a break after a maximum of four and a half hours, and in reality, will typically have a break after 3-4 hours. Since the distance covered in this time will be less than 300 km, there is the possibility to charge the truck during the driver’s break. So for most of the freight in the EU, the current battery electric trucks on the market are up to the challenge. It is expected that the range of the electric trucks available will increase to 500 km in the coming years. The key factor for an electric truck is being able to have . A strategically placed charger – ideally at a location and time when the vehicle must stop anyway – would have a significant impact on a truck’s range. However grid congestion might be a limitation for such infrastructure at scale in a short time scale.
An alternative may be a fuel cell battery electric commercial vehicle. This vehicle has proton-exchange membrane fuel cell that uses compressed hydrogen as a fuel and converts it into electricity. Hydrogen pressured at 350 bar (H35) bar is for heavy-duty vehicles and at 700 bar (H70) for light duty vehicles. The refuelling time and energy density of hydrogen is close to that of diesel that is currently used. A fuel-cell heavy truck would weigh more than a diesel one, but a battery-electric one would weigh much more than either of those. The volume of the hydrogen tanks is significant, but you don’t have your diesel tanks and the large engine is replaced by a fuel cell
Refuelling infrastructure challenge
There are several ways to refuel or charge a zero emission commercial vehicle. We start with refuelling a fuel cell battery electric commercial vehicle. This vehicle is refuelled at a hydrogen refuelling station or HRS. Light-duty fuel-cell vehicles have a 5-minute fuelling rate for filling 4 to 6 kilograms (or 8.8 pounds to 13.2) of onboard storage of hydrogen at about 1 kilogram per minute. 1 kg of hydrogen will allow you to travel 97 to 100 km. .At that 10-minute rate, Class A fuel-cell trucks (very large truck) would have enough hydrogen fuel stored to travel within a 1,100- to 1,600-km range (source).
At the end of 2021, about 730 HRSs were dispensing fuel at 350 and/or 700 bars to 880 heavy-duty trucks, 3,600 medium-duty trucks, 4,700 buses and around 42,000 cars (source IEA). Over 4,600 HRSs would need to be installed by 2030 in the NZE Scenario to support the growing fleet of heavy-duty fuel cell trucks, assuming an average nameplate capacity of over 2.5 tonnes per day (source IEA). At the end of 2022, 814 hydrogen refuelling stations were in operation worldwide. Concrete plans are already in place for 315 additional locations. Europe had 254 hydrogen stations at year end, 105 of which are in Germany. France is still second in Europe with 44 operating stations, followed by the UK (source ). The Netherlands has 10-15 HRSs depending on the sources (RVO indicates 10 and indicates 15).
The costs to build a Hydrogen Refuelling Station depends on how the hydrogen is delivered (gas, liquid or produced onsite). The former is the cheapest HRS while the latter is the most expensive one. The exact costs vary widely. The lead-time is often several years.
Charging infrastructure challenge
Ways of charging
For battery electric commercial vehicles there are four ways of charging: wired stationary charging infrastructure (depot, destination or public), battery swapping, overhead catenary charging and wireless in-road charging.
Stationary charging involves charging a vehicle at home, at the office or at the depot. This can be overnight or a moment that the vehicles is not used. The charging infrastructure for battery electric trucks differs from the charging infrastructure (with sufficient grid capacity) of electric cars. These options range from slow alternating current (AC) charging with power below (kW) to fast 150-350 (kilowatt DC) and ultra-fast direct current fast charging with power up to multiple MW (750kW-3 MW DC) (source ). The estimated charging time of fast and ultrafast is around 30 minutes. Trucks need to have larger parking spaces.
The second way of charging is battery swapping. Battery swapping technology is a system where the drained battery is taken out of the vehicle and is replaced with a fully charged battery from the battery swapping station. This minimizes the charging time and the costs of the electric vehicle could be lower (battery is a large component of the costs of a vehicle) as the battery could be part of a service agreement. So the fleet owner would only pay for the vehicle body without the battery. Battery swapping could be offered under a battery-as-a-service (BaaS) business model. There are challenges to battery swapping. First, batteries for electric vehicles are currently not standardized. They vary in shape and size and packed in the truck in different ways. Second, there needs to be a large battery inventory, a backup for each vehicle. Third, the costs to set up a battery swapping station are high. One advantage is that the battery can be charged off-peak
The third way of charging is overhead catenary charging. Overhead catenary charging allows trucks to charge while driving with electricity flowing through a pantograph connected to an overhead contact line. This technology is complementary to wired stationary charging technology, as the goal is not to electrify the entire road network. As of 2022, this technology is still at an early stage; however, several pilot projects have already been conducted, mostly in Europe and North America (source ).
A fourth way of charging is wireless in-road charging. This works by transferring electricity from magnetic coils embedded in the road to receiving coils fitted to electric vehicles. Michigan is expected to operate the first electrified roadway in 2023. The roadway’s coil segments transmit power to an EV undercarriage-mounted receiver via magnetic resonance induction as the EV moves or is parked directly above the coils. A power-management unit located either underground or above-ground near the roadside will transfer the energy from the electric grid to the roadway's copper-coil infrastructure. Both the battery size and the number of receivers connected to an EV influence the charging time. Larger vehicles can support multiple receivers (source ). But there are some challenges such as high costs to set it up, the need of technical standardization and unification of operational standards of vehicles and the impacts of radiation from high-energy wireless charging on humans and animals is currently unclear (Liu et al., 2021). Because these major challenges are to date unaddressed, wireless in-road charging is still at a very early demonstration stage and is not in commercial use (source ).
A more general consideration is that a commercial vehicle needs to be able to charge when it is connected to a charging source. For example, a vehicle could fail to connect to a charger. So chargers, batteries and electric trucks need to communicate. Interoperability regarding chargers and software means the ability to operate any software and any charging and energy hardware with each other because they are standard conforming; that is, they are compliant to publicly available technical standards published by standardization organizations (source).
Energy implications
The energy implications from e-mobility are substantial. According to electricity demand created by EV charging (public and non-public) is likely to increase from 9 TWh in 2021 to 165 TWh in 2030. This 165 TWh represents 6% of the expected EU-27 electricity consumption in 2030. The total of 165 TWh can be split into 113 TWh for cars, 23 TWh for light commercial vehicles, 26 TWh for trucks and 3 TWh for busses.
There have also been studies for the Netherlands. Netbeheer has done a study on the electricity needs for e-mobility under four different scenarios. In these different scenarios the electricity needed for e-mobility ranged from 27.5 TWh to 33.2 TWh. These numbers include all forms of mobility. E-mobility is most likely concentrated in road transport. This report was published in April 2021 so they don’t include the Fit-55 targets (source ). According to a study of Elaadnl (2022) the Netherlands needs an additional 16.7 TWh of electricity to accommodate the electric van and truck fleets by 2050 (15% of the current national consumption). This is based on a charging strategy of mostly through overnight depot charging (85% of the fleet demand) but also including public charging (15% of the fleet demand). To meet this considerably higher energy demand there needs to be large investments into the infrastructure. The Dutch government has set aside 22bn euro for infrastructure (hydrogen, heating, charging infrastructure) in the Coalition Agreement for the coming 10 years. A research paper and a report from Netbeheer show that the investment needs are substantially more.
Investments needed to support for e-mobility
According to for EU27 approximately 280 bn euro needs to be invested by 2030 in installing charging points (hardware and labor), upgrading the power grid and building the capacity for renewable energy production for EV charging. Meanwhile, a total investment of approximately 1,000 bn euro by 2050 in infrastructure is needed. This includes public and non-public charging points. To support the development of e-mobility and the rollout of electric vehicle charging infrastructure (EVCI), grid reinforcements will be necessary before connecting the chargers to the electricity networks.
The whole electricity network consists of transmission and distribution. The first is carrying high voltage electricity from power plant to a sub-station. The latter is carrying medium- and low-voltage electricity from substations to end consumers. Only the distribution systems are likely to be upgraded due to e-mobility. The most common upgrades will be transformer upgrades, modifications and network extensions at low-voltage grid, which is where the slow chargers will be connected. This is where peak power issues will be most critical and the largest congestion is expected. The expected cumulative investment into grid upgrades for EV between 2021 and 2030 have been calculated as 41 bn euro, 11% of the total annual investments of 363 bn euro in distribution system operator. The total of 363 bn euro includes investments into generic updates, electrification of buildings and houses, renewable energy generation systems and electrification of mobility. 75% of the investments (around 30 bn) are related to the upgrades of lines and transformers. The remaining 25% of investments are related to public fast chargers connected to the medium-voltage grid (source ).
The lead time and costs for cables and substations depend on the type of cable and the type of station. The lead time could be 1 to 7 seven years according to Netbeheer Nederland (source ). For example the low voltage cable has a lead time of 6 months to 1 year and medium to low voltage station also has the same lead time. According to Netbeheer 12,000-15,000 stations need to be expanded, 8,000-12,000 stations need to be added and 61,000-83,000 km of cables need to be added. This depends on the different scenarios. Most of that expansion and additions in stations are medium to low voltage stations. Roughly half of these are low voltage cables and half medium voltage cables (source ). This report is from April 2021 so the numbers have not been updated yet considering the Fit-55 targets.
Nationale Agenda Laadinfrastructuur (NAL) has indicated that the logistics sector in the Netherlands needs to consider 625 mln euro of investments by 2030 to have the charging infrastructure on own premises. These are the capital expenditures. The operational costs for the charging infrastructure amount to 1.1 bn euro until 2030. A look ahead to 2050 shows that in that case an investment of 5.2 bn euro will be required for the charging infrastructure, with associated operational costs of an estimated 7.8 bn euro. (source ).
Conclusion
The Netherlands has an ambitious target to reduce the emissions of greenhouse gasses from mobility from 31 Megaton in 2021 to 21 Megaton in 2030. The contribution of passenger cars was already discussed in our Sustainaweekly of 6 February. Commercial vehicles also need to contribute their share to reduce emissions. The targets are very clear for buses, vans and state-owned vehicles. The new proposed targets for heavy duty vehicles are ambitious if approved. There are numerous challenges to reduce emissions by commercial vehicles. These are related to the range of a battery electric vehicle, the refuelling infrastructure and the charging infrastructure including technology and grid adjustments. Therefore reaching the ambitious Dutch and European targets may prove to be difficult. It is a chicken egg story. Without the infrastructure commercial vehicles will not be able to reduce the needed emissions.