ESG Economist - The growing importance of energy storage
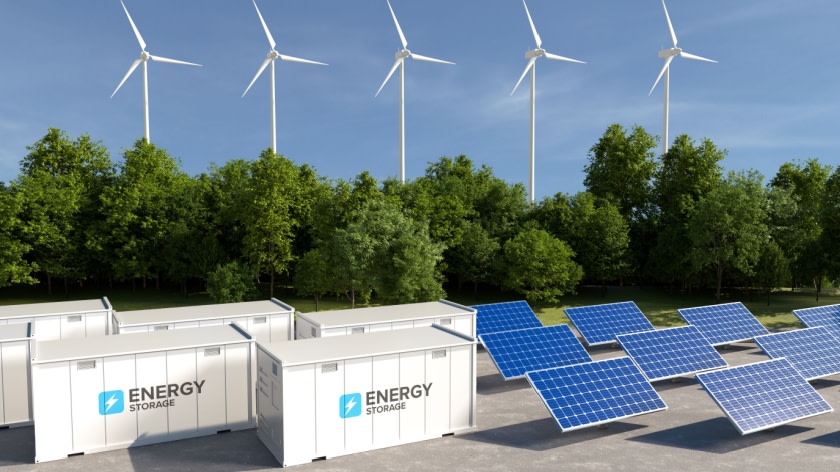
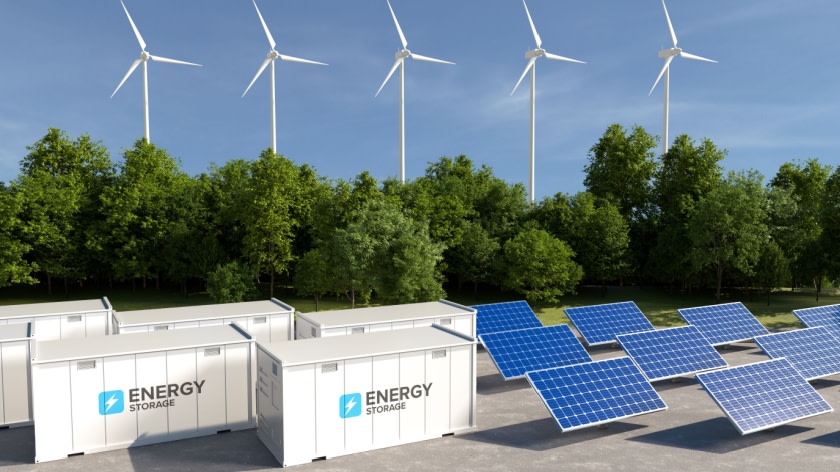
To reduce emissions at an accelerated pace over the coming years, renewables need to play an increasingly important role in the energy mix. The main problem with renewables is their intermittency, where patterns can be difficult to predict. Therefore, a way of storing the excessive renewable power for the use at a later moment is crucial. Following up on our previous report - Solutions for grid scale storage (*) - in this report, we focus on the possibilities of storage for home and businesses, also called stationary energy storage. We try to answer the following questions: What are the possibilities for stationary energy storage? What technologies are available? What are their pros and cons and what is their technical readiness? What is the policy and regulation concerning storage in the Netherlands and the EU? What is the current capacity and expected capacity for these storage technologies? How much energy is currently stored in the technologies and what is expected for 2040 and 2050? We end with a conclusion.
Energy storage is key to providing the necessary flexibility, stability, and reliability of the energy system assuming a future energy mix where renewables dominate
Energy-storage technologies are diverse. The technologies that receive the most attention: are pumped hydro storage, batteries and energy stored in hydrogen.
Batteries are more cost-effective at delivering small amounts of stored energy over a short time at high power levels. But there are different chemistries which have their own challenges.
Pumped hydro is more cost-effective at storing and releasing larger amounts of stored energy, but the technology is dependent on geography.
Hydrogen allows the energy to be stored indefinitely. But there are safety and efficiency challenges.
There are a lot of other storage technologies that could play a crucial role. One of them being thermal energy storage.
The EU and the Netherlands have policy frameworks in place for energy storage, but we think a more targeted policy to support storage would be needed.
The case for stationary energy storage
The increasingly high share of renewable energy in electricity generation will increase the need to store this energy. Energy storage is key to providing the necessary flexibility, stability, and reliability of the whole energy system. Energy storage can smooth out the intermittency of renewables and bring more balance to the grid. Without storage curtailments, negative prices would be more frequent in power markets and in consequence would decrease viability of renewable projects. Energy-storage technologies are diverse and have different features that make them suitable to provide many services to the energy system and contribute to the decarbonisation goals. In particular energy storage can support the integration of renewable energy, the electrification of the economy, and the decarbonisation of other economic sectors. Energy storage is therefore a key pillar of energy-system integration. Energy storage also makes it easier for consumers to manage their use of energy and participate in energy markets.
Furthermore, energy-storage technologies could be rapidly deployed (if the right regulatory framework and incentives exist) as a short-term measure to support energy security and help achieve the REPowerEU objectives. For example, batteries could be deployed in less than one year for stand-alone projects or in a matter of days for behind-the-meter storage. This would contribute to both energy efficiency and demand response (see more ).
What are the possibilities of storage?
Energy storage faces the challenge of converting electrical energy into a different form, which can be converted back into electrical power when demand is high. By doing this energy is lost. The round-trip efficiency (RTE) of an energy storage system is defined as the ratio of the total energy output by the system to the total energy input to the system. A high value means that the incurred losses are low. Later in this document we mention the energy efficiency of the different technologies in the tables. There are several ways to store renewable energy: mechanical, electrochemical, thermal, electrical and chemical. First, we explain the broader categories before we explain the individual technologies. We start with mechanical energy storage.
Below we explain technical readiness levels for technologies as this an important characteristic for the different technologies.
Mechanical energy storage
Mechanical energy storage technologies function in complex systems that use heat, water or air with compressors and other machinery to harness motion or gravity to store electricity. The process can be reversed to recover the energy.
The most widely deployed large-scale mechanical technical storage technology is pumped hydro-storage. It uses the gravitational potential energy of water. The potential energy is stored by pumping water to an uphill reservoir. Energy is then recovered through a hydropower turbine when the water is released downwards.
Other technologies include flywheels, compressed air energy storage and liquid energy storage (see more ). A flywheel is a mechanical device that consists of a mass rotating around an axis to enable energy storage in the form of kinetic energy. When energy is required, the flywheel transfers rotational energy to the motor that functions as a generator in this case. The rotational energy is thus converted back into electrical energy, completing the cycle. The amount of energy available and its duration is controlled by the mass and speed of the flywheel (see more ).
Compressed air technology compresses ambient air, which is then cooled and stored into a storage reservoir. During periods of high electricity demand, the pressurized air is heated and expanded in an expansion turbine driving a generator for power production (see more ).
Finally in liquid air energy storage, the charging system is an industrial air liquefaction plant where electrical energy is utilized to cool ambient air drawn from the environment until it liquifies (“cryogen”). The liquid air is then stored in an insulated tank at low pressure. When power is needed, the discharging system functions by drawing liquid air from the tank. The air is then pumped to high pressure and evaporated, producing gaseous air that can be used to drive a piston engine or turbine to generate electricity (see more ).
The table below shows the lifetime of cycles, efficiency, discharge time, technical readiness level (TRL) and pros and cons of the different mechanical energy storage systems. Most of these technologies have a high technical readiness level/are already in use and have long lifetimes. Pumped hydro storage is already widely in use, it is very efficient and has large capacity. But it is dependent on geography and has long construction lead times. Other technologies overcome some or most of the downsides of pumped hydro storage but have other disadvantages. For example, flywheels show high power density but low storage timeframes. So, this is not ideal for high storage timeframes.
Battery storage
Electrochemical energy storage technology is a technology that converts electric energy and chemical energy into energy storage and releases it through chemical reactions. During the discharging process, the energy is converted back into electrical energy. Electrochemical energy storage systems include rechargeable batteries (see more ). Particularly in small storage capacities, electrochemical storage has been popular for a long time. In the table below there is an overview of the different battery technologies that are suitable for stationary storage. Lead-acid, lithium-ion and sodium-ion batteries are relatively well-known (see more on lithium-ion and sodium ion ). Zinc-air (Zn-air) batteries and sodium-sulfur room temperature batteries are emerging rechargeable batteries.
Emerging battery technologies
We start with zinc-air batteries. In these batteries zinc oxidizes with airborne oxygen, generate energy. This unique feature, coupled with their potential for extremely high energy density, positions them as a promising option for next-generation energy storage. Additionally, they offer the advantage of being replenishable with fresh zinc, they are recyclable, and easily disposable (see more ). Although primary zinc-air batteries have been commercially available for several years, there is still a lack of rechargeable Zn-air batteries with good overall performance. They currently have limited power output, and their performance is dependent on ambient conditions such as temperature and humidity. Future commercial applications (from 2030 to 2035 on) for these batteries are for stationery (industry and utility) and consumer storage. Due to the low energy density compared to lithium-ion batteries, mobile applications are not the focus for these batteries.
Next to zinc-air batteries there are sodium-sulfur room temperature batteries. They also have relatively low technical readiness level (4), but they could be an interesting alternative stationary storage solution especially in the long run (later than 2035). Sodium-sulfur high temperature batteries (temperature range 300-350°C) are already available for stationary storage. In this technology thermal management system is crucial.
The table below shows the different electrochemical energy storage technologies and their different characteristics. Most of the technologies have efficiency levels equal to pumped hydro energy storage and flywheels and they also have high technical readiness levels. But these stationary batteries have considerably shorter lifetime/lower cycles. Batteries are more cost-effective at delivering small amounts of stored energy over a short time at high power levels. Pumped hydro is more cost-effective at storing and releasing larger amounts of stored energy.
Thermal energy storage
Thermal energy storage includes all the technologies that allow the capture and storage of thermal energy for later use. This form of energy has great potential especially in the industrial and building sector to replace fossil fuels with renewable-energy solutions in heating systems. Most of the industrial energy demand consists of process heat which can be generated by renewable energy sources and thermal storage. There are several ways to store heat: sensible heat storage, latent heat storage and thermochemical heat storage.
We start with sensitive heat storage systems. These systems raise or lower the temperature of a liquid of solid storage medium to store and release thermal energy. Energy storage temperature is from <0°C to 2,400°C for a duration that can range from minutes up to months (see more ). There occurs no change in phase. Adequate thermal insulation is required to guarantee that the system works well. The storage materials include water, molten salts, graphite, rocks. This thermal storage has the highest technical readiness (7-9) compared to latent heat storage (4-9) and thermochemical storage (4-6).
Latent heat storage systems accumulate thermal energy thanks to the medium's latent heat which means the heat required to change phase without a change of temperature. This can store heat at temperatures ranging from <0°C to 1,600°C for a duration of hours to days (see more ). The temperature is constant, the charging or discharging of the tank is related to a change of medium's phase (see more ). During the change of phases, for example from solid to liquid, the material absorbs energy, which then remains bound in the material as so-called latent energy. Latent heat storage systems can store energy without major losses over a longer period (see more ).
In thermochemical storage the energy is stored as part of an endothermic reaction (a process that absorbs heat from its surroundings) instead of an increase in temperature. Thermochemical heat storage operates in two ways: chemical reactions and sorption processes. In the former, energy is stored as the heat of reaction or reversible reactions. The latter stores thermal energy either through adsorption (physical bonding) or absorption (uptake/dissolution of a material). These technologies can store thermal energy at temperatures ranging from <0°C to 900°C for a duration of hours to days.
The table below shows the different thermal energy storage and electrical energy storage technologies and their characteristics.
Electrical energy storage
Next to mechanical, battery and thermal energy storage there is also electrical energy storage. Examples are capacitors, supercapacitors (less for stationary storage, TRL of 5-8) and superconducting magnetic energy storage. A capacitor is a device that stores electrical energy by accumulating electric charges on two closely spaced surfaces that are insulated from each other. Energy storage in a capacitor is based on maintaining an electric field in which the energy is stored. It can take a shorter time than a battery to charge up and it can release all the energy very quickly. Capacitors store and distribute energy in short bursts while batteries store and distribute energy linearly. They are commercially available.
Superconducting magnetic energy storage is a technology that stores energy like a battery. The AC (alternating current) power from an external source is first converted to DC (direct current) power. The main difference between AC and DC lies in the direction in which the electrons flow. In DC, the electrons flow steadily in a single direction, while electrons keep switching directions, going forward and then backwards in AC. The DC power is then passed through the superconducting wire to generate a large electromagnetic field, which is ultimately used to store this energy. Superconducting materials have zero electrical resistance when cooled below their critical temperature. Because of the zero electrical resistance the magnetic field is perfectly efficient. Once the superconducting coil is charged, the DC in the coil will continuously run without any energy loss, allowing the energy to be perfectly stored indefinitely until the system is intentionally discharged. This high efficiency allows end-to-end efficiencies of over 95% (see more ). This technology has great potential but is not commercially available yet at large scale.
Chemical energy storage
Finally, there is chemical energy storage. Chemical energy storage is energy stored in the form of chemical fuels that can be readily converted to mechanical, thermal or electrical energy for industrial and grid applications (see more ). Examples of chemical fuels are methane, methanol, ammonia and hydrogen. Hydrogen allows the energy to be stored indefinitely. But there are challenges to hydrogen (we are publishing a major note on hydrogen soon). To produce renewable hydrogen 20-30% of energy is lost. The hydrogen must then be compressed and stored losing another 10%. Finally, another 30% is lost when converting hydrogen into electricity. As a result, the round-trip efficiency is around 30-40%, much below that of other storage technologies.
Hydrogen can function as chemical energy storage, but it can also be transformed into methane and ammonia. Hydrogen and captured CO2 can be used to produce synthetic methane, which can be stored or used within the existing natural gas grid. Methanol can be easily stored and transported compared to other fuels. It can also be converted into a variety of other chemicals and has a potential as transportation fuel. Ammonia is another potential energy carrier. It can be formed from hydrogen and nitrogen. There is an existing infrastructure due to its widespread use as fertilizer, but it has serious safety issues. The technical readiness levels of chemical energy storage ranges from 6 to 9.
The graph below shows a summary of the storage technologies based on discharge duration and typical power capacity. These characteristics and the other characteristics in the tables above determine the choice of storage technology.
EU 2030 climate targets
We have discussed the case for energy storage, the technologies and characteristics of each technology. In this section we focus on what framework the EU (and subsequently the Netherlands) has in place to support energy storage.
EU policy for energy storage
Market and regulatory conditions in each Member State affect and determine the deployment of energy storage. The main drivers observed in Member States have been specific ancillary services and supporting schemes. In addition, several Member States have added a definition of energy storage into their legislative framework, reducing the risk that energy storage might be treated as both a consumer issue and a producer issue. Some Member States have also adopted strategies or national targets in their National Energy and Climate Plans (NECPs) for energy storage for 2030 and 2050 to incentivise the deployment of energy storage at national level.
The European Commission adopted in March 2023 a list of recommendations to ensure greater deployment of energy storage (see more ). It addresses the most important issues contributing to the broader deployment of energy storage. EU countries should consider the double 'consumer-producer' role of storage by applying the EU electricity regulatory framework and by removing barriers, including avoiding double taxation and facilitating smooth permitting procedures. Indeed, there was a proposal to revise the Energy Taxation Directive, and this proposal includes a specific provision to end the double taxation of energy storage. According to the recommendation, EU countries should also identify flexibility needs across different timescales and ensure that system operators assess these needs when planning network developments. In terms of financing, it suggests increasing the long-term visibility and predictability of revenues to facilitate access to finance, for example by allowing storage operators to receive remuneration for certain services that they currently provide. EU countries should consider instruments, such as competitive bidding procedures (in line with state aid rules) to achieve the necessary flexibility and improvements in the design of certain parameters within capacity mechanisms.
The Recommendation was accompanied by a (SWD/2023/57) (see more ) which looked at the role and application of storage in the energy transition, emphasising the need for flexibility, reliability and stability. It provided some global outlook for storage deployment and an overview of best practices.
Facilitate energy storage
Several EU regulations and initiatives already facilitate the development and deployment of energy storage as a key technology to support the decarbonisation objectives of the European Green Deal. For example, the REPowerEU plan specifically highlights the importance of energy storage in ensuring flexibility and security of supply in the energy system. The revised Renewable Energy Directive also contains specific provisions facilitating the deployment of electric vehicles and encouraging demand-response and energy storage as a source of flexibility, including for thermal energy storage. A further element of the Fit-for-55 package is the proposed revision of the Energy Efficiency Directive, also encouraging demand-response and energy storage to increase efficiency. On the top of these, the revision of the Energy Performance of Buildings Directive encourages: (i) the effective control, storage, and use of energy; and (ii) the installation of smart recharging infrastructure in buildings, which is particularly important since this is where electric vehicles park regularly and for long periods of time.
Funding energy storage
Public funding contributes to the financing of energy-storage technologies, addressing certain investment barriers (regulatory, economic and technical) to make it more attractive to invest in projects. There are specific support and financing tools available to incentivise the deployment of energy storage in the EU, financed from both EU’s long-term budget as well as from the NextGenerationEU (NGEU) package. Cohesion policy will continue to support Member States, regions, and local authorities to invest in energy storage through the available funding of the European Regional Development Fund (ERDF), the Cohesion Fund (CF) and the Just Transition Fund (JTF). Energy storage is also eligible for support under the EU Renewable Energy Financing Mechanism (REFM) when deployed in combination with new renewable-energy capacity. Other instruments and funds contribute in some specific territories to the financing of storage, such the Innovation Fund (IF) and the Modernisation Fund (MF). In addition, specific support and financing tools at national level help the deployment of energy storage projects. The revised guidelines on state aid for climate, environmental protection, and energy (CEEAG) have introduced a set of new provisions facilitating support for energy storage. In addition, the state aid General Block Exemption Regulation (GBER) is under revision to further facilitate public support for the EU’s green and digital transition, including relevant provisions for energy-storage projects in the revised proposal (see more ).
Expected net installed capacity
The increasingly volatile nature of the electricity generation sources will require deployment of storage solutions. Daily storage needs are currently met by pumped hydropower (PHS) and increasingly by batteries. By 2030, the PHS capacity will grow by from currently 45 GW to 64 GW in the baseline scenario. Due to geographical conditions, its growth potential in Europe is limited. However, there is still growth potential if there is an extension of pumped hydropower and small hydropower projects. Storage solutions complemented in time with electrolysers and more flexible demand will play a key role to integrate the different components of the energy system, allowing for a full decarbonisation and the full deployment of, notably, renewable primary energy sources. The growth of the installed electrolyser capacity is expected to accelerate significantly after 2030, reaching already between 40 to 70 GW in 2035 and between 528 and 581 GW in 2050 in the policy scenarios. This development will go along with the decarbonisation of the gas system, which may necessitate partial repurposing of gas infrastructure (see more ).
The Netherlands policy framework for energy storage
The Netherlands also has a framework in place. The Netherlands is focusing on electricity storage by investing in battery innovations and incentivising batteries in large-scale solar parks. It also allows solar energy to be used if the sun does not shine and relieves the electricity grid.
The Netherlands promotes hydrogen production at sea, as well as energy exchange with North Sea countries, allowing long-term storage and exchange of energy. The National Hydrogen Programme was launched in 2022. This public-private programme focuses on unlocking the supply of renewable hydrogen, developing the necessary transport, storage and import infrastructure, working with various sectoral programmes, and facilitating ongoing initiatives and projects. Hydrogen transport, storage and import projects are included in the Multi-Annual Programme for Infrastructure for Energy and Climate (MIEK), through which the Government manages these projects.
Furthermore, the regulatory framework allows everyone, subject to specific conditions, to use the electricity system, for example through the deployment of battery storage or the introduction of solar production by households (see more ).
Energy storage roadmap
The Energy Storage Roadmap maps out the actions to be taken to promote energy storage, appropriate to its expected role in the future energy system, up to 2035 and beyond. The Energy Storage Roadmap looks at all forms of energy storage, divided into electricity, molecule and heat storage. The Energy Storage Roadmap contains three main elements (see more in Dutch):
An analysis of the current state of energy storage in the Netherlands and an overview of expected developments in the future.
An inventory of actions for successful roll-out of energy storage in the Netherlands until 2035, with specific action holders and targeted deadlines.
Grants
There are no subsidies for home batteries in the Netherlands yet. As with individuals, there are no specific subsidies for batteries for large-scale energy storage for businesses. In specific situations, business can deduct from tax the purchase of a home battery through the Energy Investment Allowance (EIA). This scheme has been extended by 5 years through 2028. With the EIA, you can deduct 40% of the investment amount of the battery from your taxable profit. So, you pay less corporate or business tax. The EIA is for companies that pay income or corporate tax in the Netherlands. The business asset you purchase is listed in the Energy List. In this case, it is specifically about batteries for the stationary storage of sustainably generated electrical energy. The Energy List targets mainly lithium-ion batteries and saltwater batteries. The battery costs minimal 2,500 euros.
The Demonstratie Energie- en Klimaatinnovatie (DEI+) also applies for energy storage. You receive a grant to test an innovative technique in a pilot project or to demonstrate it in a demonstration project. For example, you introduce an application of a device, system or technique that reduces CO2 emissions that is new to the Netherlands. Your innovation must help reduce CO₂ emissions at low cost. This helps us in the energy transition (the transition from fossil fuels to sustainably generated energy) (see more ).
European Commission proposal for climate targets beyond 2030
Net installed capacity under the different scenarios
The European Commission set out a proposal for climate targets beyond 2030. It formulated three different scenarios. Target option 1 is a net GHG reduction target of up to 80% (S1). This is compatible with a linear trajectory of net GHG emissions between the existing 2030 target and the 2050 climate neutrality. Target option 2 is net GHG target reductions in 2040 of at least 85% and up to 90% (S2). Target option 3 is a net GHG reduction target in 2040 of at least 90% and up to 95% (S3). The Commission recommended a target somewhere in between S2 and S3. Next to these scenarios there is a LIFE or more sustainable lifestyles scenario.
In this proposal there are scenarios for net installed capacity (in GW) and the quantity of energy that can be stored (in TWh). A gigawatt-hour (or TWh) is used to measure quantity of energy. While a gigawatt expresses power, that is to say the energy flow produced or consumed at time t. We start with net installed capacity of the storage technologies.
Whatever target is in the end decided, storage capacity would need to be ramped up significantly. Pumped-hydro storage capacity would need to grow from 50 GW in 2020 to 75 GW in 2040. Deployment of battery storage would need to accelerate after 2030, from 100 GW to 135-200 GW in S1-S2-S3 in 2040 enabling mostly the daily and weekly storage of electricity.
Meanwhile electrolyser capacity would need to increase from 30 GW in 2030 to 185-300 GW in 2040. The measures accompanying LIFE reduce the need for flexibility, in particular of electrolyser capacity.
Power-to-X technologies provide additional flexibility in the future by adjusting production levels to match the pattern of intermittent electricity generation. Installed power-to-gas and power-to-liquid capacities remain relatively low. Power-to-X describes the conversion of renewable electricity from wind, water or solar as primary energy into an energy carrier. Between 2040 and 2050, batteries and pumped storage are projected to remain relatively stable, while electrolysers show additional growth (see more page 35). The graph below shows how much storage capacity is needed under the different scenarios.
Stored energy under the different scenarios
Above we showed the net installed capacity under the different scenarios. We now focus on the energy stored under the different scenarios. Currently energy is stored mainly into pumped hydro storage. The electricity stored in pumped hydro is projected to grow from 25 TWh in 2020 to 35-50 TWh in 2040. Batteries are expected to surpass pumped hydro storage as the main source of providing storage between 2025 and 2030, reaching 160 TWh in 2030. By 2040, electricity stored in electrolysers (10-70 TWh) plays a minor role in providing storage to the electricity system than that stored in batteries (200-240 TWh), as the available electrolyser capacity to produce hydrogen will be used in sectors other than the power sector. In 2040, methane storage, i.e., clean gas, will play a minor role covering 4-15% of stored electricity in S1-S2-S3. The measures of LIFE are projected to result in a slight reduction in stored electricity in 2040. The four scenarios result in different compositions of stored electricity by technology. Methane storage displays a crucial uptake in S3 where it reaches 50 TWh or 15% of all stored electricity in 2040. The lower use of methane storage in S1 is compensated by hydrogen, which covers 20% of the total stored electricity, in contrast to S3, where it only accounts for 4%. Until 2050, batteries remain the dominant electricity storage covering 63% of all stored electricity. The amount of total stored electricity remains stable between 2040 and 2050 despite the uptake of renewables in the electricity mix. The graph below shows the stored energy across scenarios (see more page 37).
Taken all the policy and scenarios into account the eurozone and the Netherlands have policy frameworks in place for energy storage, but we think a more targeted policy to support storage would be needed to reach the capacity and storage goals under the different scenarios.
Conclusion
To reduce emissions and to be net-zero by 2050 renewables play a crucial role. The main problem with renewables is intermittency, where patterns can be difficult to predict. Therefore, a way of storing this energy for later use is needed. Energy storage is key to providing the necessary flexibility, stability, and reliability of the whole energy system. Energy-storage technologies are diverse. The technologies that receive the most attention: are pumped hydro storage, batteries and energy stored in hydrogen. Batteries are more cost-effective at delivering small amounts of stored energy over a short time at high power levels. But there are different chemistries which have their own challenges. Pumped hydro is more cost-effective at storing and releasing larger amounts of stored energy, but the technology is dependent on geography. Hydrogen allows the energy to be stored indefinitely. But there are challenges to hydrogen such as safety and efficiency.
There are a lot of other storage technologies that could play a crucial role. One of them is thermal energy storage. Thermal energy has great potential especially in the industrial and building sector to replace fossil fuels with renewable-energy solutions in heating systems. Most of the industrial energy demand consists of process heat which can be generated by renewable energy sources and thermal storage.
The eurozone and the Netherlands have policy frameworks in place for energy storage, but we think a more targeted policy to support storage would be needed to reach the capacity and storage goals under the different scenarios.
See download for the hyperlinks in graphs