Applications of carbon capture in the energy transition
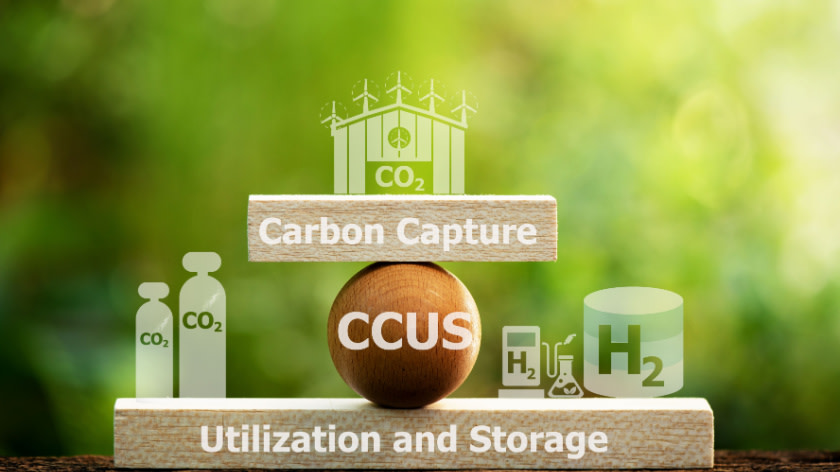
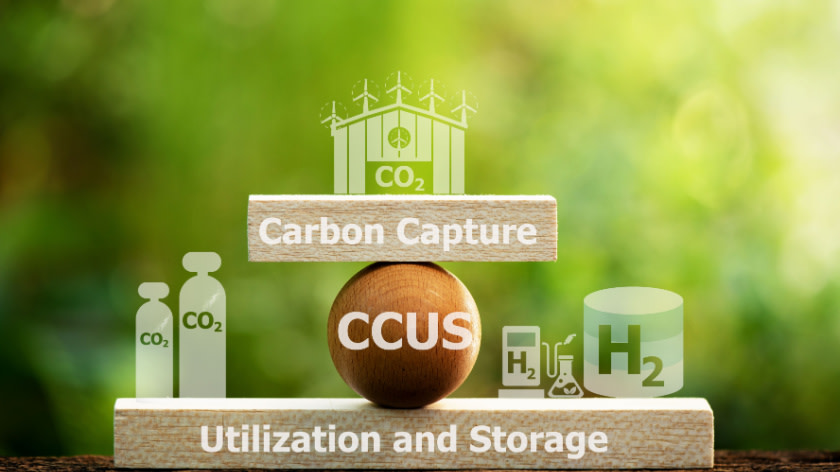
Carbon capture and storage (CCS) is essential for the energy transition, but remains still a very small share among CO2 reduction technologies. In this piece, we dig into the different layers of carbon capture technologies, and discuss how the various techniques play a crucial role in improving the technologies and bringing the overall CCS costs down. Currently CCS is one of the more expensive technologies to decarbonize.
Carbon capture holds a very small share among technologies to reduce CO2 emissions, but a crucial one
Most common carbon capture technologies are post-combustion, pre-combustion and oxy-fuel
The technique used (within the technology) to separate CO2 is equally important
The costs of both the technologies and techniques need to be reduced to scale up CCS use
Introduction
The goal is reaching net zero by 2050and staying within the carbon budget aligned to a pathway to stay below 2°C degree above pre-industrial levels and pursue efforts to limit the temperature increase to 1.5°C above pre-industrial levels. There are several ways to achieve this goal. First, limiting and mitigating CO2 and other greenhouse gas emissions. Second, capture emissions from combustion. Third, trap CO2 and other emissions from the atmosphere. To reach the goal a combination of these three ways are necessary. Up to now our articles on technologies have mainly been centred around the first point of reducing emissions. Examples are the use of more sustainable fuels, battery technologies and solar technologies. Currently carbon capture is only done on a relatively small scale. In 2022 the world emitted 37 Gt CO2 and only 46 Mt CO2 was captured via CCUS or 0.1%. For 2030, the IEA expects emissions to decline to 24 GtCO2 and the total capture capacity (including operational, under construction and planned) to reach 321 Mt CO2 or 1.3% in a net-zero scenario (see graphs below). Even though it is a sharp increase in capacity compared to 2022, it is still a very small share of CO2 reduction compared to the total.
In this note we focus on technologies that capture emissions from combustion. This is the CC (carbon capture) of CCUS (carbon capture, utilisation and storage). In future notes, we will focus on technologies used to store these emissions, to utilise the captured CO2 and the technologies that trap CO2 and other greenhouse gasses from the atmosphere. We first start with the different carbon capture technologies and then the techniques used in these technologies.
Carbon capture technologies
Carbon capture technologies involve capturing CO2 from the combustion of fossil fuels. For carbon capture there are several layers of technologies. The first layer includes technologies of the point of capturing. The second layer of technologies or techniques includes the materials that are used to capture the CO2. In this layer material specific characteristics are the crucial variables. We start with the first layer of technologies. There are three main technologies used to capture carbon from fossil fuel combustion: post-combustion, pre-combustion and oxy-combustion.
Post-combustion technology
Post-combustion captures CO2 from burning fossil fuels or biomass before it enters the atmosphere. Post-combustion CCS generally entails capture from flue gases with low CO2 concentrations. In the case of solid fuels, CO2 concentrations are generally below 15% (IPCC, 2005; IEAGHG 2013) at low pressure. This carbon capture technology is the widely preferred option for retrofitting existing power plants. Post-combustion CO2 has higher power use and compression costs due to the low concentration of CO2 in the flue gas. A significant advantage of the post-combustion carbon capture technology is its maturity compared other existing carbon capture alternatives. There are a variety of techniques (the second layer) that can be used in post-combustion to separate CO2 from the flue gas, including absorption, adsorption, membranes and other techniques. We will go into more details in the second part of this report. In the table below are the different CCS technologies with technical readiness level (TRL), uses, pros and cons and which techniques these technologies use (second layer).
Mobile carbon capture
Usually CCS technologies are used at large stationary sources as at power generation and industrial facilities. But more and more research is done of using CCS for mobile sources. This is called mobile carbon capture. It refers to the process of capturing CO2 at the point of emission — namely, from the vehicle while it is in motion — before it is released into the atmosphere. Research and tests have been done for trucks and ships. If this application of this technology develops furthers, it could be a solution for the hard to abate transport sub-sectors such as heavy duty vehicles, maritime shipping and inland shipping.
Pre-combustion technology
Pre-combustion capture refers to removing CO2 from fossil fuels before combustion is completed. In gasification processes a feedstock (such as coal) is partially oxidized in steam and oxygen/air under high temperature and pressure to form synthesis gas. The syngas is then processed in a reactor, which converts the CO to CO2. The concentration of CO2 in this mixture ranges from 15% to 50%. The high CO2 concentration in the syngas significantly enhances the absorption efficiency, leading to the formation of a fuel that can be less harmful to the environment (see ).
Oxy-fuel combustion technology
Oxy-fuel with post combustion (or oxy-fuel) refers to the replacement of air, either in the boiler or the gasifier, with pure oxygen (O2). Using purified O2 helps eliminate unwanted by-products present in air, and also increases the CO2 purity of the resulting syngas or flue stream, making carbon capture more effective. Oxy-combustion overcomes the technical challenge of low CO2 partial pressure normally encountered in conventional coal combustion flue gas by producing a highly concentrated CO2 stream (~60 percent). Partial pressures refers to the relative concentrations of differing substances in gas phase. Partial pressure influences CO2 capture costs, because it affects the size of process equipment, capture plant energy requirements, and applicable capture technologies. The higher the pressure, the easier CO2 will be captured, and the process will be cheaper due to less energy consumption and smaller capture equipment. The partial pressures is an important variable on how to capture CO2. Whereas post combustion capture works well with existing installations, oxy-combustion is an opportunity for new infrastructure.
Chemical looping technology TRL5-6
Chemical looping is an advanced form of combustion that does away with the need for an expensive air separation unit. In its place it uses a solid-state oxygen carrier. It relies on combustion or gasification of coal in a Nitrogen-free environment. However, rather than using an air separation unit, chemical looping involves the use of a metal oxide or other compound as an oxygen carrier to transfer O2 from air to the fuel. Because this is not an end of pipe technology, it is important, that the used oxygen carrier is capable of handling impurities in the fuel feed and to know how the fuel conversion performance and lifetime of oxygen carrier particles is influenced. Chemical looping could also be classified as pre-combustion technology.
How to separate the CO2
As indicated above there are a variety of techniques (the second layer) that can be used in post-combustion to separate CO2 from the flue gas, including absorption, adsorption, membranes and other techniques. Some of these techniques are also used in the other CCS technologies such as pre-combustion. Below we explain the different techniques.
Absorption (solvents) techniques
In this technique, a liquid solvent, known as the absorbent, is used to selectively dissolve CO2 from the gas mixture.
Conventional solvents rely on chemical absorption to remove carbon dioxide but there are also physical solvents.
We start with chemical solvents. Chemical solvents usually contain an amine that will react selectively with carbon dioxide. The industrial gas is fed into an absorber, where the solvent will react safely and quickly with carbon dioxide. The CO2-rich solvent will then be transferred to a regenerator, where the temperature will be raised to around 120 degrees Celsius. This increase in temperature reverses the carbon dioxide absorption reaction, producing a pure CO2 gas and fresh solvent. The CO2 is captured before being sent for geological storage or onward use. The fresh solvent can be returned to the absorber to start the cycle all over again (see ). This regeneration process is highly energy intensive. There are two generations of commercially-available amine solvents. First-generation solvents such as monoethanolamine (MEA). MEA is most widely used for CO2 absorption, due to its high selectivity, quick reaction and low cost. However, it is also sensitive to impurities and requires desulfurization and denitrification of the flue gas to work effectively. Second-generation solvents include improved blends of sterically-hindered alkaloamines and amino acids that require lower regeneration temperatures and are more resistant to degradation. However, they cost more than MEA and do not perform as well as MEA. By adopting amine technology, 85-95% of CO2 can be captured from the flue gas (IPCC, 2005). It is a mature technology but energy requirements for capture and compression of CO2 lead to higher fuel consumption, hence net CO2 reductions achieved are lower than the capture rate. Traditional amine solvents has a technical readiness level (TRL) of 9. Other chemical liquid solvents could have a TRL 2-7.
When using physical solvents for CO2 capture, there is no chemical reaction so the driving force for CO2 absorption is the solubility of CO2 in the solvent which generally increases with pressure and decreases with temperature in accordance with Henry's law. Henry'slaw is a gas law which states that the amount of dissolved gas in a liquid is directly proportional to its partial pressure. Modern pre-combustion CO2 capture systems use solvents to selectively absorb CO2 from gas mixtures using the physical absorption process. This is because of the relatively high CO2 partial pressure in (see ). They hold CO2 in solution through physical mechanisms rather than chemical reactions, and as such the solution of CO2 is weaker and easier to break by heating, so less energy (or heat) is needed. Examples for physical solvents are Selexol and Rectisol (TRL 9). The most frequently used and mature process is the Selexol process. This has a technical readiness level of 9 according to Global CCS Institute.
Adsorption (sorbents) techniques TRL7-9
The process of adsorption involves molecules in liquids and gases adhering to solid surfaces by weak van der Waals interactions. Van der Waals forces are driven by induced electrical interactions between two or more atoms or molecules that are very close to each other. Adsorption is another separation technique which is slightly different from absorption because adsorption includes specific creation of physical and chemical connection between CO2 and the surface of the adsorbent. Sorbents and solvents are similar, but in solvents the CO2 is absorbed into a liquid whereas for sorbents the CO2 is adsorbed onto solids. After the sorbent captures and releases the CO2, the sorbent can be used again. Selection criteria for this sorbent include a large surface area, high regeneration capability, and high selectivity. It is possible to achieve CO2 adsorption by changing the pressure or temperature of a saturated sorbent. Physical solid sorbents used in carbon capture are mesoporous silica, metal-organic frameworks (MOFs), carbonaceous materials (activated carbon, graphene, carbon nanotubes), polymers, calcium oxide, (CaO), clay materials, alkali metal carbonates, magnesium oxide (MgO), layered double hydroxides, alkali silicates.
This technique has the advantage of lower regeneration energies compared to solvents but these sorbent technologies are generally less developed than solvents. The use of solid sorbents can be for post and pre-combustion technologies. Solid adsorbents have a TRL of 5-9 depending on the sorbent. Metal organic frameworks (MOF) are possible solution for mobile carbon capture mentioned above.
Calcium looping (CaL) is a second generation carbon capture technology. Calcium looping is a CO2 capture scheme using solid CaO-based sorbents to remove CO2 from flue gases, It is a promising CO2 capture technology. It also produces a useful by-product for the cement and lime industry. This technology has a technical readiness level of 6-7 (2020, see ).
Membranes TRL 4-6
Membrane separation uses a semipermeable membrane or barrier to physically separate CO2 from other flue gases. It is a promising next-generation technology for post-combustion CO2 capture. It is the selective transport and separation of CO2 through membrane from flue gas under the driving force of pressure difference. It can significantly reduce energy consumption and chemical footprint relative to mature amine scrubbing that is in widespread use. Nevertheless, despite many studies commercially viable membranes are not widely implemented at a large scale (see ). It is potential to be more energy- efficient than the aforementioned methodologies when treating CO2 feed content >10 %. But a direct comparison of energy consumption between amine absorption and membrane separation technologies is difficult due to the different energy source required (electricity for membrane and heat for absorption). Membrane separation does not involve hazardous chemical storage, handling, or disposal, but they have lower separation efficiency than those mature technologies. The highest TRL level for CO2 capture from flue gas using membranes is currently at pilot-scale. Can be used in pre- and post-combustion capture.
Other techniques to separate CO2
Cryogenic Carbon Capture (CCC) is a highly innovative CO2 capture technology. CCC process separates CO2 from light gases in essentially any continuous process. It cools the gases to the frost or desublimation point of CO2 (−100 to −135 °C). Desublimate is to change directly from gas to a solid. It separates and pressurizes the solids, and warms all streams to produce a CO2-depleted stream at ambient pressure and a pure (99+%) pressurized liquid CO2 stream typically to about 150 bar, both at ambient temperature. The process also recovers all gas moisture and most gas impurities less volatile than CO2 (NOx, SOx, mercury etc.) in separable streams. The process also has several major additional advantages. For a start, it is a retrofit technology that does not need steam or any modification of existing equipment. Furthermore, it recovers water and nearly all pollutants in addition to CO2 from the flue gas. It also enables highly efficient and cost effective energy storage at grid scale and on time scales of minutes. But one disadvantage of CCC is the accumulation of CO2 frost reduces the efficiency of the capture process. The process must be periodically shut down to regenerate the heat transfer surface and collect CO2 that has been frozen out of exhaust gases. The TRL is 6.
Hot Potassium Carbonate or HPC capture is a post-combustion technology that bypasses the degradation issues of amines. It starts with the cooling and compression of the flue gases to enable enhanced efficiency of CO2 absorption in the absorber. The HPC solvent captures the CO2 from the flue gases in the absorber and is subsequently regenerated in the desorber. It seems to capture of over 90% of CO2.It allows for lower energy consumption in the capture process than comparable technologies.
Conclusion
There are several ways to reduce emissions. First, limiting and mitigating CO2 and other greenhouse gas emissions. Second, capture emissions from combustion. Third, trap CO2 and other emissions from the atmosphere. Carbon capture technologies currently have a small share of reducing CO2. It is likely that its share will rise substantially but will be relatively low compared to other technologies and the overall emissions in 2030. However, carbon capture technologies are crucial to compensate for the hard to abate sectors. Capturing CO2 can be done by post-combustion, pre-combustion and oxy-fuel combustion. Moreover the various techniques used in these technologies also play a crucial role to improve the technologies and to bring the costs down to use CCS more widescale and/or in mobile situations. Currently CCS is one of the more expensive technologies to decarbonize.