ESG Economist - Geothermal energy has great potential but there are challenges
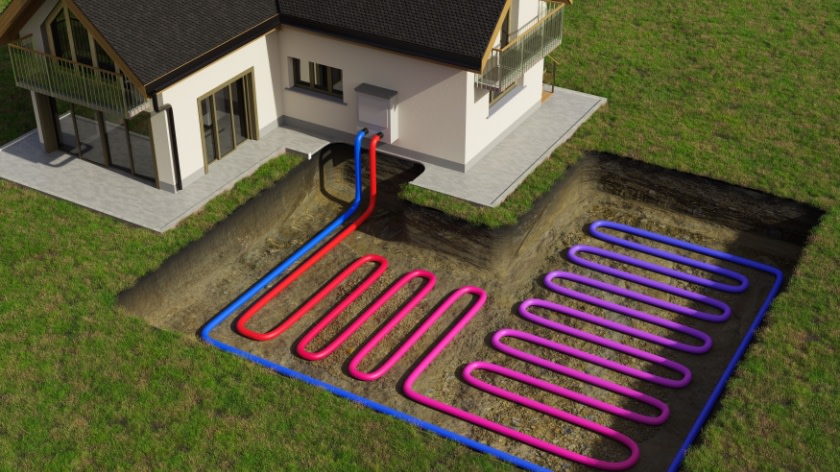
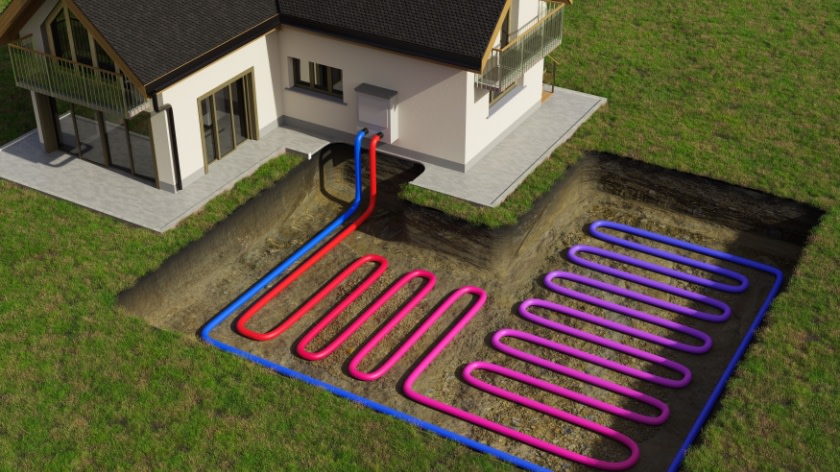
Geothermal energy can be used for electricity generation and heating and has only a fraction of the life cycle emissions compared to burning fossil fuels. Geothermal energy currently meets less than 1% of global energy demand but it has enormous potential.
Unlike solar and wind, which have an intermittency challenge, geothermal energy can provide around-the-clock electricity generation, heat production and storage
But geothermal energy also has challenges
The heat from the Earth is infinite while a geothermal system is for 20 or 30 years
Geothermal energy runs the risk of triggering earthquakes due to alternations in the Earth’s structure
Geothermal energy as a decarbonizing technology is expensive compared to the other technologies. To make it economically feasible subsidies, higher fossil fuel prices and/or higher ETS prices are needed
Introduction
Geothermal energy is a technology to reduce emissions for heating and electricity production compared to technologies that use fossil fuel. It is a technology currently not widely used but it has great potential. According to the IEA, geothermal energy generation currently meets less than 1% of global energy demand (see more here). Data from Eurostat show that geothermal electricity generation is less than 0.25% of gross electricity generation in EU27 and less than 0.1% of the total installed electricity capacity. The graphs below show geothermal electricity generation and installed capacity in the EU as a whole.
Unlike solar and wind which have an intermittency challenge, geothermal energy can provide around-the-clock electricity generation, heat production and storage. So, it is the renewable energy source unlike any other. According to IEA geothermal power can operate at their maximum capacity throughout day and year. On average, global geothermal capacity had a utilisation rate over 75% in 2023 compared with less than 30% for wind and less than 15% for solar PV ().
In the Netherlands geothermal energy is used for heating but not used for electricity generation yet. In this publication we focus on the potential of geothermal energy in the Netherlands. We explain what geothermal energy is. Then we show the composition of the subsurface of the Netherlands, what composition is good for geothermal energy and what determines a good potential for geothermal energy. We then explain the different geothermal systems to use heat from the Earth. We also focus on the marginal abatement costs of geothermal energy. We end with a conclusion.
What is geothermal energy?
Geothermal energy is heat (thermal) derived from the Earth (geo). It is the thermal energy contained in the rock and fluid that fills the fractures and pores within the rock of the Earth's crust. There are two sources of heat: ground source energy and geothermal energy. Ground source heat is the heat stored in the ground up to 400 meters deep. This heat is solar-derived heat. Geothermal heat is heat derived from the Earth, it comes from within the Earth, and it is deeper than 500 meters.
What are the sources of geothermal heat? First the heat is left over from when our planet was formed. Since its formation, the Earth has been losing heat into space. So, it is the heat that has not been lost yet. The inside of the Earth is still very hot; it is between 4,400°C-6,000°C.
Second, heat is caused by denser core material sinking to the centre of the planet. This is called frictional heating. Frictional heating is a form of energy dissipation, which is responsible for the increase in temperature at the contact region of the sliding surfaces .
Third, if materials crystallize from liquid to solid it results in heat. Crystallization is the process by which solids form, where the atoms or molecules are highly organized into a structure (=crystal). Whenever crystallization takes place, it involves reduction in motion of atoms or molecules, which is released in form of heat.
Finally, heat is also caused by the decay of radioactive elements. This is called decay heat. During radioactive decay mass is converted into energy and an important part of this energy is converted into heat (). About 50% of the internal heat of the Earth is originated from radioactive decay.
While Earth’s internal heat is the energy source for processes like plate tectonics and parts of the rock cycle, it provides only a fraction of a percent to the Earth’s average atmospheric temperature. Plate tectonics explain the function of the uppermost layers of the planet. Overall, Earth’s interior contributes heat to the atmosphere at a rate of about 0.05 watts per square meter, while incoming solar radiation adds about 341.3 watts per square meter ().
So, heat stored in the Earth can be derived from solar (ground source) or derived from the Earth itself (geothermal heat). The temperature within the Earth is increasing with depth. On average the temperature is increasing in continental crust by 20-30°C for every kilometre depth. You can harvest heat from areas with high heat flows such as volcanic zones or from sedimentary rocks such as sandstone and limestone of considerable thickness.
Netherlands suitable for geothermal energy
For some countries such as the Netherlands the surface is flat. But in the Dutch subsurface there are quite some structures. The composition of the subsurface is important to determine if there is potential for geothermal energy. There are several factors that determine if a layer is suitable for geothermal energy. These factors are depth, thickness of the layer, porosity and permeability. The depth is important because the deeper the borehole the higher the temperature. The thickness of the layer indicates the geothermal potential. The thicker the layer the higher the potential but the potential is higher if the layer is between clay layers that close off this layer (water stays within the layer). Furthermore the material needs to have good porosity and permeability. Porosity and permeability allow for efficient fluid flow, which is essential for geothermal heat extraction. The ideal soil for geothermal energy is composed of 50% solid, 25% air and 25% water. In the Netherlands there are four suitable formations . The different layers can be targeted for different types of geothermal energy (shallow, mid-deep or deep, see more on this below). The Netherlands has great potential for different types of geothermal energy because of suitable formations being present.
Technologies
In this section we focus on the different geothermal technologies. Above we indicated that the subsurface is not even and that there are potential layers at different depths. The four suitable formations in the Netherlands are Rotliegend sandstones, Triassic sandstones, lower Cretaceous and Upper Jurassic sandstones and finally Tertiary sandstones. To investigate if the geothermal energy is a possible solution for heating and/or electricity, one needs to investigate if the subsurface at a certain place has one or more of these suitable formations. Next to this it is important what the aim is for using geothermal energy. Questions we need to ask ourselves:- Will the geothermal energy be used for one home, multiple homes, industry or electricity production? - Is there a need for heating, cooling or both? - What are the legal and environmental aspects that need to be considered? If there is an overview of suitable formations, aim of the geothermal energy, heating /cooling or both, regulations and environmental issues then the technology can be chosen. Below there are more details about the different technologies.
We mentioned that source of the heat in the Earth can be solar-derived, or Earth derived. For solar-derived heat there are shallow geothermal systems and for Earth-derived heat there are deep geothermal systems (see graph below). The sun is only able to heat the shallow layers of the earth. Solar-derived heat has lower temperatures and needs heating with heat pumps to further increase the temperature from around 14°C to the desired level. Earth-derived heat have heating without the need of heat pumps. Furthermore, very deep geothermal systems are used for electricity production process heat . The steam comes from the reservoirs of hot water and this steam rotates a turbine that activate a generator which produces electricity . The graph below shows the different geothermal technologies for ground source heat and geothermal heat.
Shallow geothermal systems
Shallow geothermal energy or ground source heating is a system of extracting renewable heat energy from the ground, concentrating it and using it to supply heat and domestic hot water. The boreholes don’t go deeper than 400 meters. For ground source heating there are several technologies to use the heat: boreholes thermal energy storage (BTES), Aquifer Thermal Energy Storage (ATES) and mine water energy. A ground source heat pump (GSHP) is needed to increase the temperature to the desired level.
Borehole thermal energy storage (or BTES)
This technology uses an array of boreholes (narrow shafts bored in the ground) to store excess heat in shallow geological environments (). The borehole heat exchanger (or BHE) is inside the borehole. It is a devise to extract geothermal heat from the shallow rocks . The boreholes can have a vertical loop, horizontal loop, slinky loop or pond loop. A loop is a plastic pipe shaped like a U. The picture below shows a system with a horizontal loop. The temperature is generally low between 10-14°C. The ground can’t cool too much otherwise the system is not useful.
The system transfers and stores excess heat from the ground via the fluid (water-glycol medium). The fluid is a water-glycol medium or water with a nontoxic anti-freeze. Vertical loop systems have boreholes up to 150 meter and are for smaller projects and houses. These are closed loop systems. This means that no groundwater is pumped and there is no impact on groundwater, if there is it is small. There are no specific hydrogeological conditions required. The downside is that these systems require a lot of space.
A closed (horizontal) loop works as follow. the Sun warms the Earth (1). The cold fluid pumped out of the house (2) extract the heat from the ground (3). The heated fluid is carried into the house (4). The heat pumps heat the water further and transfer the heat to the home (5). The cold fluid is pumped out of the house.
Aquifer Thermal Energy Storage (ATES)
In an Aquifer Thermal Energy Storage natural ground water is used to store the thermal energy. An aquifer is an underground layer of water-bearing material, consisting of permeable or fractured rock, or of unconsolidated materials. It obtains energy directly from the water by pumping it through a heat exchanger (in most cases there is a heat exchanger before the heat pump itself). These collectors are vertical systems where water is most sourced by pumping groundwater from a borehole or a well. Once the heat energy has been extracted, the groundwater can then be injected back into the ground using a re-injection well (this way it is in balance). This graph below shows this process. During the summer warm water is injected, and the cold water is pumped (see picture below). The injected water can only be max 25°C. In the winter the process is reversed. This is an open loop system and is always in balance .
This system can have separate warm and cold wells (doublet system) or cold and warm wells in one borehole (mono well system). Doublets are used for large scale projects and mono well systems for small(er) scale projects. The table below shows the difference between open and closed geothermal systems.
Mine water thermal energy
Next to BTES and ATES it is also possible to extract the heat stored in flooded mines. Warm mine water is extracted from a borehole. A heat pump extracts heat from the mine water and uses it to heat homes. The hot water circulates in separate loop to homes. When the heat is extracted the mine water is returned to the mines to be reheated. This technology can be used in countries that have a lot of flooded mines. Mine water has temperatures between 10-25°C.
Mid deep geothermal systems
Above we discussed that ATES systems have maximum injection temperatures of 25-30°C. These are low temperature ATES systems or LT-ATES. There are also high temperature ATES systems. High temperature ATES systems involve seasonal storage of residual heat. It can be directly applied to a district heating network without using heat pumps. These have storage temperatures above 50°C usually between 70-90°C. They use aquifers with a depth between 150-500 meter.
Deep geothermal systems
High temperature geothermal energy involves boreholes to deep layered aquifers with higher temperature than 60 °C or granite layers with temperature above 130°C. The former can be directly applied to district heating without using heat pumps and it is for large scale projects meaning more than 2,500 houses. Deep geothermal energy with boreholes to granite layers are used for electricity production. Deep geothermal wells are up to 5 km deep. Systems with boreholes deeper than 4,000 meters are called ultra deep. Where temperatures are suitable for energy production, a reservoir is created by injecting water or another fluid at high pressure to create or expand fractures in the rock. This is enhancing its permeability. These systems are called enhanced geothermal systems. They can be open-loop or closed-loop. The injected fluid absorbs heat from the rock as it flows through the reservoir. This hot fluid is then pumped back to the surface. For power generation the heated fluid passes through a heat exchanger or turbine system at the surface to generate electricity or provide direct heating. Afterward, the cooled fluid is re-injected into the reservoir, maintaining reservoir pressure and ensuring sustainability (see picture on geothermal energy systems above) .
Challenges for geothermal energy
Geothermal energy has a lot of advantages, such as low life cycle emissions (low emission costs) and that it is a reliable non-intermittent source of energy for a long period. But there are also several disadvantages. First even if warmth from the Earth seems infinite, a geothermal system is not. In shallow geothermal systems, harvesting heat from the Earth results in a lower temperature over time in that location. This means that at a certain point the borehole or systems does not provide enough heat anymore, so another location needs to be sought. For deep geothermal energy system, the cooler water is also injected in a well. For Earth to rewarm this water still will also take more than 10 years.
Second, another point to keep in mind is that there is continuous movement in the subsurface visible by earthquakes and volcanic eruptions. During subsurface operations there are changes in subsurface pressures, temperatures, mass and volumes. Changes in the stress situation can result in the fracturing of the reservoir and/or fault slip . Failure of faults and rocks is potentially seismic. There are concerns that geothermal activity may result in seismicity. Induced seismicity refers to earthquakes that are caused by anthropogenic activities. A report published by TNO, an applied science and technology organisation, shows that despite low to medium seismogenic potential for geothermal targets in the Netherlands, felt seismicity associated with geothermal operations cannot be excluded, even if mitigation measures are implemented. Absolute guarantees that felt seismicity will be absent cannot be given due to uncertainties in geology and interaction of operations with that geology, considering the uncertainty of locations and stress changes at faults. Mitigation measures such as local seismic monitoring combined with a traffic light system can be successful approaches to reduce seismic risk in these cases ). Traditional traffic light systems are based on decision variables (e.g. seismic magnitudes) and on threshold value on which actions must be taken (based on expert judgment and regulations, ).
Third, geothermal energy has high upfront costs and considerable operational costs. The table below shows global weighted average total installed costs in USD per kW. For geothermal energy these are one of the highest; only concentrated solar power is higher. But geothermal energy has the highest capacity factor. This is a measure of how much energy is produced by a plant compared to its maximum output. So how often the plant is running at maximum power. The table also shows that geothermal has one of the highest levelized cost of electricity (LCOE).
The LCOE mentioned in the table above is used to calculate the marginal abatement costs in EUR per tonne for different geothermal energy systems. We calculate the avoided emissions compared to the Dutch and European energy mix. As indicated above it has very low life cycle emissions (LCE). As a result, a lot of emissions can be avoided. The LCA, avoided emissions and the LCOE are the basis for the marginal abatement cost calculation. The result in USD is converted into EUR to be able to compare it with the average EU ETS price of 2024. As the table below shows geothermal energy needs a much higher ETS price to become economically feasible. Subsidies would also help to make geothermal become economically feasible.
Dutch government policyThe Dutch government is taking several measures to encourage geothermal energy. The main measures are as follows. First the government supports companies drilling for geothermal energy with the Geothermal Energy Risk Coverage Scheme (). If a drilling is unsuccessful, the company receives compensation. Second, with the Sustainable Energy Production and Climate Transition Incentive Scheme (), the government supports the production of renewable energy, including geothermal heat. Third, the investigates where the subsurface is suitable for geothermal heat extraction. Fourth, through companies and organisations are investigating whether it is possible to extract heat deeper than 4,000 metres from the subsurface. This geothermal heat at ultra-deep depth is suitable for light industry. In the Netherlands, there is no experience with ultra-deep geothermal heat yet. In , the government and industry work together to reduce CO2 emissions from greenhouse horticulture. Among other things, by informing horticulture companies about the use of geothermal heat.
With these measures, the government wants to achieve more geothermal projects. Which will make its production cheaper and cheaper. The goal is to generate 15 petajoules of sustainable energy from geothermal heat by 2030. That is roughly the average gas and electricity consumption of 2.2 million households.
Conclusion
In this report we focused on the potential of geothermal energy. Geothermal energy can be used for electricity generation and heating and has only a fraction of the life cycle emissions compared to burning fossil fuels. Unlike solar and wind which have an intermittency challenge, geothermal energy can provide around-the-clock electricity generation, heat production and storage. So, it is the renewable energy source unlike any other. The Netherlands has four suitable formations that can be targeted for different types of geothermal energy. But geothermal energy also has challenges. First a geothermal system is for 20 or 30 years. Second, the potential of despite low to medium seismogenic potential for geothermal targets in the Netherlands, felt seismicity associated with geothermal operations cannot be excluded, even if mitigation measures are implemented. Third geothermal energy as a decarbonizing technology is expensive compared to the other technologies. To make it economically feasible subsidies, higher fossil fuel prices and/or higher ETS prices are needed. So geothermal energy has great potential in the Netherlands and the Dutch government has in place policy to lower the costs of Geothermal energy, but challenges remain.
Gunatilake, 2024. Geothermie. Geothermie - Nederlandse Ondergrond, VU Amsterdam).
Gunatilake, 2024. Geothermische energie. Geothermie - Inleiding, VU Amsterdam).
Drijver, 2024. Geothermie. Ondiepe geothermie, VU Amsterdam).
Drijver, 2024. Geothermie. Ondiepe geothermie, VU Amsterdam).
Andeweg, 2024. Geothermie. Deep Geothermal Energy, VU Amsterdam).
Andeweg, 2024. Geothermie. Deep Geothermal Energy, VU Amsterdam).